Insights
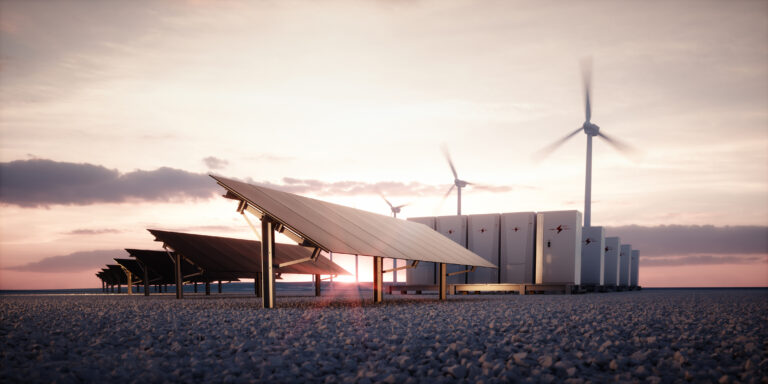
July 17, 2020
If you read our previous article on electric vehicle (EV) battery recycling, you know that just 5 percent of lithium-ion batteries are recycled. With millions of retired batteries (RBs) from EVs coming offline over the next decade, an important question is whether the RBs can enjoy a second life before ultimately being recycled. EV manufacturers typically replace batteries when their state of health (SOH) is around 70 to 80 percent – SOH representing the ratio of current capacity to that of a fresh battery.1 This is due to decreasing performance (slower acceleration, shorter range) and energy efficiency that occurs as the battery is repeatedly cycled. The wide range of SOH for RBs, in practice 70 to 90 percent, is a result of the lack of SOH estimates on EVs, as well as environmental conditions and difficulty in determining the battery capacity needed by the EV owner.2
The suitability of RBs for second-life application depends heavily on their usage during first life. If batteries face demanding conditions, it is likely they will face a relatively decreased capacity during their second life. This declining SOH curve is known as a battery’s aging curve. The curve is non-linear and the SOH eventually hits what is known as an aging knee, where the decline in SOH decreases at a relatively greater pace (Figure 1). Aging knees are particularly evident in battery cells with cobalt chemistries – those batteries with high energy density, such as NCM chemistries.
Figure 1. Bacon–Watts Knee Prediction Model 3
Before determining the suitability of RBs for second-life use (either reuse, remanufacture, or repurpose), they must first be disassembled and tested:
An important part of the repurposing of RBs is following electrical safety regulations – in particular, UL 1974 (Standard for Evaluation for Repurposing Batteries), which provides requirements for sorting and grading of batteries that are intended for repurposing. UL 1974 includes requirements for parts of the repurposing process, including quality control, facility safety, examination, and sorting. The breadth of testing helps ensure systems built with SLBs are built to the same [safety and reliability] standards as systems built with new batteries.4
There are five broad applications of repurposed SLBs:
Over the short term, SLBs may be more suitable for commercial and residential applications rather than utilities given the safety risk large scale configurations present. In China, large-scale SLB energy storage systems were banned for the time being by the National Energy Administration in 2021.5 The most common application for repurposed SLBs are stationary battery energy storage systems because requirements for range, energy density, and power capability are high for EV and mobile applications – passenger EV batteries are designed for high range and high-power density rather than cyclic lifetime.
The lifetime of an SLB depends on its application and can range from 6 to 30 years. Research from Casals et al suggested via simulation that SLB lifetime could extend beyond 30 years for fast charging EV stations, reach almost 12 years for self-consumption and transmission delay, and last 6 years for area regulation.6
The opportunity that SLBs present relates to their attractive price. While some literature and websites quote the price of SLBs as 35 to 60 percent of new batteries, Neubauer et al’s analysis suggests a more reasonable assumption is a price ranging from 50 to 70 percent of new batteries.7 8 However, even with a lower price, it remains to be seen whether SLBs can repeatedly provide an improved cost-benefit profile when compared to fresh batteries, given factors such as system replacement, installation, transport, downtime, etc.
Additional challenges facing RBs include transportation, as batteries are classified as hazardous material. Furthermore, the battery management system (BMS) from the EV battery is likely not appropriate for second-life use, meaning each application needs an appropriately engineered BMS. Battery modules also vary from one vehicle to another, and specific modules might not be well suited for SLB applications. Lastly, batteries that perform well at initial end of life might be able to be reused or remanufactured into a new vehicle battery pack, reducing flow to repurposed SLB applications. In fact, researchers have questioned the retirement of batteries at SOH around 80 percent. Saxena et al found that 80 percent SOH can meet the daily needs of 85 percent of US motorists.9 As well, Martinez-Laserna et al questioned retiring batteries in EVs at SOH of 70 to 80 percent.10
The labour-intensive disassembly and inspection process for RBs also presents challenges. Disassembling and inspecting battery cells can prove to be difficult and costly, yet the nascent industry lacks standardized methods for safety assessment.11
Currently, there is a lot of on-going research into the field of battery aging mitigation and lifetime expansion. If BMS advances and other innovative techniques can extend the life of EV batteries, all else equal, that longer first life would mean a lower beginning SOH for SLBs.
Second life system manufacturers could benefit from coordinated development with EV manufacturers so that the whole lifecycle of the EV battery is taken into consideration. This could allow for the standardization of manufacturing and improved battery quality tracking, streamlining the conversation of RBs into SLBs. Whether this takes place and on what timeline are anyone’s guess. Given the margin compression automakers (less Tesla) are facing on EVs, there is likely little appetite to standardize manufacturing to accommodate SLB considerations over the medium term when costs are so out of control. Ford, for example, was losing $36,000 on each EV it sold in Q3.12
The budding SLB industry presents challenges and opportunities. From the perspective of a battery’s lifecycle, we believe batteries should enjoy a second life (condition permitting) before being recycled, and we are hopeful that companies can make SLBs safe and economic.
1 S. Saxena et al., “Quantifying EV battery end-of-life through analysis of travel needs with vehicle powertrain models,” J. Power Sources, vol. 282, pp. 265-276, May 15 2015.
2 L. C. Casals, B. A. García, and L. V. Cremades, “Electric vehicle battery reuse: Preparing for a second life,” Journal of Industrial Engineering and Management, vol. 10, no. 2, pp. 266-285, 2017.
3 Paula Fermín-Cueto, Euan McTurk, Michael Allerhand, Encarni Medina-Lopez, Miguel F. Anjos, Joel Sylvester, Gonçalo dos Reis, Identification and machine learning prediction of knee-point and knee-onset in capacity degradation curves of lithium-ion cells, Energy and AI, Volume 1, 2020
4 Martin F. Börner, Moritz H. Frieges, Benedikt Späth, Kathrin Spütz, Heiner H. Heimes, Dirk Uwe Sauer, Weihan Li, Challenges of second-life concepts for retired electric vehicle batteries, Cell Reports Physical Science, Volume 3, Issue 10, 2022
5 https://www.idtechex.com/en/research-report/second-life-electric-vehicle-batteries-2023-2033/924
6 L. Canals Casals, B. Amante Garcia, and C. Canal, “Second life batteries lifespan: Rest of useful life and environmental analysis,” J. Environ. Manage., vol. 232, pp. 354-363, Feb 15 2019.
7 Of, not less than. For example, if the price of a new battery was $100/kWh, the price of an SLB would be $70/kWh.
8 J. Neubauer, K. Smith, E. Wood, A. Pesaran Identifying and Overcoming Critical Barriers to Widespread Second Use of PEV Batteries (2015)
9 S. Saxena et al., “Quantifying EV battery end-of-life through analysis of travel needs with vehicle powertrain models,” J. Power Sources, vol. 282, pp. 265-276, May 15 2015
10 E. Martinez-Laserna et al., “Technical Viability of Battery Second Life: A Study From the Ageing Perspective,” IEEE Trans. Ind. Appl., vol. 54, no. 3, pp. 2703-2713, May-Jun 2018
11 B. Jiang, H. Dai, and X. Wei, “A Cell-to-Pack State Estimation Extension Method Based on a Multilayer Difference Model for Series-Connected Battery Packs,” IEEE Trans. Transp. Electrif., vol. 8, no. 2, pp. 2037-2049, Jun 2022.
12 https://insideevs.com/news/693626/ford-cuts-ev-investment-after-losing-36000-usd-every-ev-sold-q3/